1. Overview of electricity
Electricity is the fastest growing end-use in energy, and indeed, to fight climate change, we actually want electricity to dominate energy end-use globally. In other words, we want to increase electricity's share of end-use energy to 100% or as close to it as we can get.
This is partly because we want more people to benefit from the electricity-provided services we take for granted, like electric lights and TVs and air conditioning. Air conditioning is a major "adaptation" to living in a hotter climate. The World Health Organization describes heat waves as "among the most dangerous of natural hazards."
We also want electricity's share of total energy use to grow because there is an emerging consensus in the clean energy community that the best and cheapest way to build a 100% clean energy system is to electrify everything. That is, we need all buildings (including heating, cooling and cooking), transportation and industrial energy use to be converted to electricity (with some exceptions).
Globally, 39 percent of electricity comes from low-carbon sources like nuclear energy or renewable energy (including solar, wind, hydropower, wave and tidal, and some biomass). This means 61 percent comes from fossil fuels.

2. Sources of clean electricity
We are loosely categorizing solar photovoltaics (or PV), wind turbines, and geothermal sources as relatively “uncontroversial” solutions because virtually everybody agrees we need to accelerate the deployment of these generating technologies to have a shot at staying within 1.5 C of average warming.
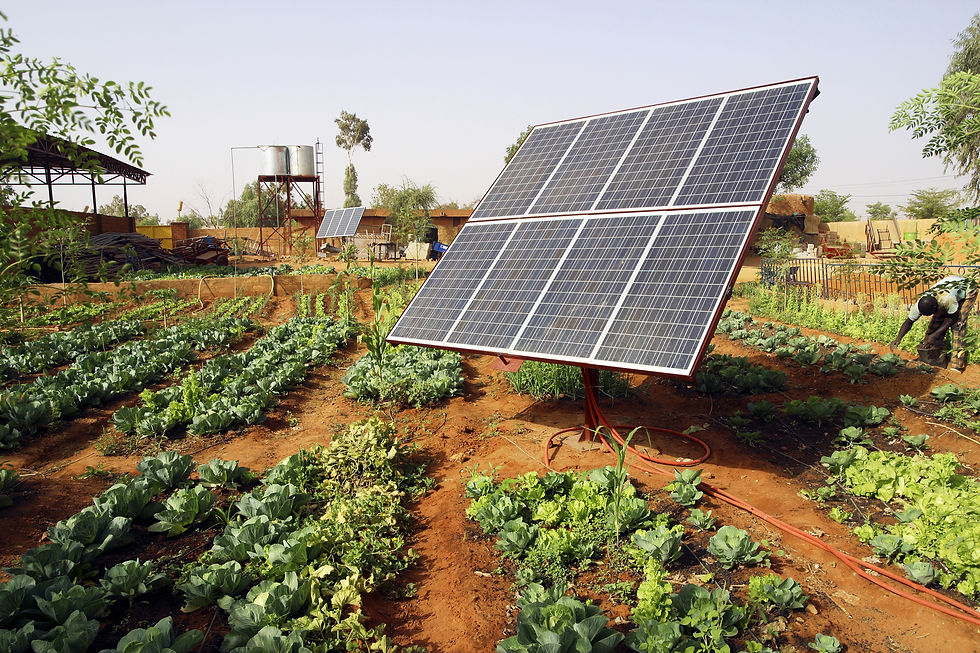
Nuclear energy, hydropower, and biomass energy are the more “controversial” bunch. Depending on your assumptions, politics, and even personal preferences, you may have strong views for or against all of these.
Solar photovoltaics
Solar PV systems convert photons of light directly into electricity. These panels, which you typically see on rooftops and in solar farms, are different from solar thermal systems that convert light and infrared radiation into thermal energy (heat). There are different types of solar PV technologies. The vast majority of solar cells, which are the building blocks of solar modules, are made of silicon.
Despite an unprecedented pandemic, global solar capacity doubled in three years from 2018, bringing the world’s solar fleet to one Terawatt capacity in April 2022. According to the IEA, we need to triple our capacity additions of solar PV by 2030 to be on track to achieve net-zero emissions from energy by 2050.
Correspondingly, multi-silicon solar module prices have plummeted by 90 percent over the past decade, though they are now rising slightly. And while the typical solar panel's efficiency is in the 15 to 20 percent range—meaning they convert that much incoming light energy to electricity—new technologies in commercial labs report efficiencies up to 47 percent.
Solar's market growth has been achieved through a combination of factors, including governmental support, R&D investment, private sector investment, and clean energy advocacy by industry and environmental groups.
Solar PV systems can be “distributed” (e.g. on individual rooftops) or “utility-scale” (large solar farms). These days you can even find floating solar farms—and solar panels on actual farms, too, in the emerging world of agrivoltaics.
Ownership models can also vary: Utilities, solar companies, individuals and communities can all own solar PV systems, depending on local policy and regulatory contexts.
Wind turbines
Wind turbines today are of a staggering scale: The 13MW GE Haliade-X turbine, described in this article in the New York Times from January 2021, features a blade that is 107 meters in length. A single turbine is capable of powering approximately 12,000 homes. Alongside are some fun photos of what it's like to transport a standard wind turbine blade by road.
You can also buy small wind turbines, scaled for a community or even just an individual home.
According to the Global Wind Energy Council, “Today, there is now 743 GW of wind power capacity worldwide, helping to avoid over 1.1 billion tonnes of CO2 globally – equivalent to the annual carbon emissions of South America.” Globally, wind is the primary non-hydro renewable technology, generating almost as much electricity in 2020 as all other renewables combined.
Onshore wind was one of the cheapest new sources of electricity in 2020, and offshore wind power has seen price reductions exceeding 67% over the last 8 years. According to the IEA, we need to increase wind capacity additions four-fold by 2030 to be on track to achieve net-zero emissions from energy by 2050. Wind turbines can be “onshore” (on land) or “offshore” (out on the ocean). These days they can even be floating and double as places for coral reefs to grow.
Geothermal energy systems
Geothermal energy is simply energy derived from the earth's crust.
Its affordability depends on the underlying geology, though the technology for deeper drilling is advancing fast, greatly expanding the choice of places where this type of energy can be tapped.
The advantage of geothermal energy is it can simultaneously provide energy for electricity and heating/cooling applications. Geothermal District Heating is gaining popularity in Europe; these systems can provide for the energy needs of homes, commercial buildings and even some industry, for entire neighborhoods and even cities, as discussed in this podcast. So far, geothermal technologies haven't managed to scale at a pace comparable with wind and solar, but that could change.
Geothermal technologies can also include ground-source heat pumps, which use steady shallow-earth temperatures to heat and cool buildings and sometimes provide hot water—and which are starting to take offglobally.
Biomass energy
Biomass energy is energy from organic matter—both plant materials and animal waste. Plant-based biomass energy is considered a "sustainable" source of energy because plants absorb carbon dioxide from the atmosphere, and when burned, emit the same amount of carbon dioxide back into the air. So in theory, biomass energy is carbon "neutral." But the reality is quite different, as we will discuss in the next slide. About 10% of global primary energy comes from biomass. Many rural people around the developing world rely on wood, charcoal, animal dung, and other biomass to provide energy for household cooking and heating, and small-scale rural industrial processes, like brick-making and food processing. Modern bioenergy sources include:
Solid fuels like torrefied wood pellets or briquettes
Biofuels, which include:
First-generation fuels from food crops (ethanol and biodiesel)
Second-generation fuels from lignocellulosic biomass
Third-generation fuels from algae
Fourth-generation fuels like electrofuels and photobiological solar fuels—these are still experimental systems.
The E.U. started backing away from biodiesel once it discovered that the fuel was derived from palm oil plantations created by cutting down tropical rainforests and critical biodiversity habitats in Southeast Asia. Other stories, like the one depicted alongside, began to draw wider attention to this problem. More recently in the U.S., a new five-year study of the impact of corn ethanol—a widely used fuel additive in the U.S.— found that it has led to increased fertilizer use, water pollution, and likely at least 24 percent more emissions than gasoline.
The World Resources Insitute reports that increasing biofuel cultivation takes precious land away from food production and that ambitious biofuel targets could significantly increase the “food gap” between crop calories available vs. those needed in 2050. Other studies like this one have also documented the food-energy trade-off. While some bioenergy proponents suggest we can use "marginal land," evidence suggests that there is less marginal land than we think: Many poor people rely on such lands for grazing and/or food cultivation.
A related problem is the "indirect land use" question: This is a situation in which biofuel cultivation simply displaces other types of cultivation, like food crops, to other lands, in the same country or overseas, that could encroach into former rainforests or other natural habitats, and result in a net increase in greenhouse gas emissions. This has been researched in Brazil; however, it is complicated and difficult to assess the extent of this impact.
A type of carbon removal approach called BECCS, or bioenergy with carbon capture and storage, featured in IPCC scenarios for 1.5 C, is also shown to be unsustainable from a land-use perspective.
While large-scale use of biofuels has fallen out of favor, biomass energy based on crop residues and/or animal waste can be sustainably used on a small scale, and can often be an excellent solution for rural energy needs. Many rice-growing regions produce a lot of rice husk; this can become a fuel source for small gasifiers that generate power. Biogas is also a feasible option for some rural communities, but it has constraints—you need sufficient crop residues and animal waste year-round for it to be an effective solution. However, there are places where it has been proven to work, like in the Indian state of Chattisgarh.
Nuclear energy
Nuclear energy can, in theory, be generated via two processes: fission or fusion. All commercial nuclear power is produced through fission, which involves the splitting of atoms to release energy. Fusion is the process of combining or fusing atoms—it's how the energy in the sun is generated. No fusion reactor exists, though it remains the holy grail of nuclear research.
Nuclear energy is clean as far as carbon emissions go, as it does not emit greenhouse gases during operation. There is a relatively small amount of carbon emitted during construction of plants (mainly due to the use of concrete), and in mining and transporting the fuel, but this is comparable to the lifetime emissions of wind and solar production. However, nuclear waste management continues to be a challenge in some countries, like the U.S., for a range of political and historical reasons. Other countries, like France, Canada, and Sweden have solved this problem in a variety of different ways. France produces 70% of its electricity from nuclear energy. Globally, however, nuclear energy is losing popularity. This is partly due to costs—nuclear plants take considerable time and funding to build, and wind and solar energy, even with storage, are outcompeting nuclear power in many places. Indeed, critics argue nuclear is "well past its sell-by date," and say "it is clear that renewables will do the heavy lifting of our energy transition."
Again, it's important to emphasize that context matters. France is planning to build 14 new nuclear reactors by 2050. Meanwhile, Germany and California both recently announced closures of nuclear power plants—this has been very controversial from a climate perspective as the power they produced has been replaced by gas-fired electricity, at least in part. Nuclear advocates point out that nuclear energy is firm, dispatchable power, unlike variable wind and solar, but as we will soon cover in this class, we have other solutions for grid balancing, and cost issues still persist: One over-hyped project in the U.S. has experienced staggering cost overruns and delays.
And then there is the big question of the very small risk of a very catastrophic event—remember Fukushima? Or do you envision beautiful, modular, clean, and safe new technologies, such as the one pictured in the image alongside?
New technologies are, indeed, getting some attention, though the jury is out on whether any of these can be built at a scale and generate power at an affordable cost in the near future, when we need to double down on emissions reductions. And small modular reactions may, if anything, intensify concerns about managing and disposing of nuclear waste.
Still, there are some interesting developments in the works: Next-Gen Nuclear Plant and Jobs Are Coming to Wyoming, and large clean energy investment firms like Bill Gates' Breakthrough Energy are investing in next-generation fission technologies and fusion. The U.S. Office of Nuclear Energy has a brief overview of advanced reactors systems to watch.
Hydropower
While large dams have provided very cheap electricity and other vital development services (like irrigation water) to millions around the world, they are also the cause of significant deforestation, biodiversity loss, and forced human displacement. The costs of mega dams can outweigh the benefits.
Note that large dams are not necessarily climate-saving—in tropical regions, large dams can contribute more emissions than fossil fuel power plants for decades, as submerged forests decompose releasing potent methane. In spite of their downsides, over 500 new dams are planned or under construction in protected areas around the world.
Mini- and micro-hydro projects are an entirely different story. These smaller-scale systems can power local mini-grids and provide other services (e.g. water reservoirs for the dry season). These systems typically make the most sense in hilly regions where perennial streams and waterfalls exist.
Commentaires